Inviterte
foredrag til 8. Norske Polymer- og kolloidvitenskapelige
Vårmøte
Novel
Amphiphilic Block Copolymers
Made
by Controlled Free Radical Polymerization
André
Laschewsky
Universität
Potsdam, Institut für Chemie Karl-Liebknecht Str.24-25, 14476
Potsdam-Golm
laschews@rz.uni-potsdam.de
and
Fraunhofer Institut für Angewandte Polymerforschung FhG-IAP ,
Geiselbergstr.69, 14476 Potsdam-Golm (Germany),
laschewsky@iap.fraunhofer.de
Amphiphilic block copolymers
are a
most intriguing species of amphiphiles.1-6 However, the preparation of
such
block copolymers had been considered for long to be the "holy grail"
of polymer synthesis, as generally, delicate living polymerization
methods had been
necessary. These methods are mostly cumbersome, very sensitive against
impurities, and have only low tolerance to functional groups, in
particular to
such that are hydrophilic, consequently limiting the synthesis of
amphiphilic
block copolymers to few systems. This situation changed dramatically
with the
advent of the methods of controlled free radical polymerization (CFRP)
since
2000,7-9 which are much more tolerant
of
functional groups. This created a booming research field in the past
decade,
and has made numerous polymers with so far unusual - or even unknown -
block
structure and of unusual architecture accessible.3, 4
This contribution will first
introduce to the strategies, opportunities and difficulties encountered
in the
synthesis of amphiphilic block copolymers by CRP, in particular by the
RAFT
(reversible addition fragmentation chain transfer) method. Then, the
making of
classical polymer architectures will be addressed. Finally, unusually
complex
block copolymer amphiphiles made accessible by virtue of this technique
will be
exemplified, highlighting amphiphilic stimulus-sensitive systems,
triphilic
ternary block copolymers, and dual brush giant surfactants.10-16
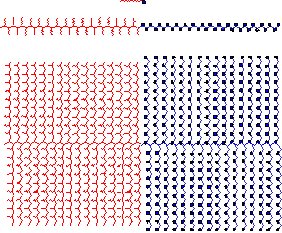 |
Scheme 1. Comparison of
the
architecture of a standard surfactant, a macrosurfactant, and a giant
surfactant (from top to bottom). The left (~~~)
and the right side (·) represent the
hydrophobic and hydrophilic
elements, respectively.
|
References
- I. W. Hamley, Block
Copolymers in Solution: Fundamentals
and Applications. John Wiley & Sons Ltd: Chichester,
England, 2005.
- S.
Garnier; A. Laschewsky; J. Storsberg, Tenside
Surf. Det. 2006, 43,
88-102.
- J.-F. Lutz, Polym.
Int. 2006, 55,
979-993.
- A. Blanazs; S. P. Armes; A. J. Ryan, Macromol. Rapid Commun. 2009, 30,
267-277.
- M. A. Cohen Stuart, Colloid
Polym. Sci. 2008, 286,
855–864.
- I. C. Reynhout; J. J. L. M.
Cornelissen; R. J. M. Nolte, Acc. Chem.
Res. 2009, 42, 681-692.
- K. Matyjaszewski; T. P. Davis, Handbook of Radical Polymerization. John
Wiley and Sons, Inc.: Hoboken, 2002.
- K. Matyjaszewski; ed., Macromolecular
Engineering: Precise
Synthesis, Materials Properties, Applications. Wiley-VCH:
Weinheim, 2007; Vol. 1.
- V. Percec; ed., Chem.
Rev., vol.109 (11), thematic issue
on: Frontiers in Polymer Synthesis 2009.
- M. Arotçaréna;
B. Heise; S. Ishaya; A. Laschewsky, J. Am.
Chem. Soc. 2002, 124,
3787-3793.
- K. Skrabania; J. Kristen; A.
Laschewsky;
Ö. Akdemir; A. Hoth; J.-F. Lutz, Langmuir
2007, 23, 84-93.
- M. Mertoglu; S. Garnier; A.
Laschewsky;
K. Skrabania; J. Storsberg, Polymer 2005, 46, 7726-7740.
- K. Skrabania; H. v. Berlepsch; C.
Böttcher; A. Laschewsky, Macromolecules
2010, 43, 271-281.
- A. Laschewsky; J.-N. Marsat; K.
Skrabania; H. v. Berlepsch; C. Böttcher, Macromol.
Chem. Phys. 2010, 211,
215-221.
- D. Zehm; A. Laschewsky; M.
Gradzielski;
S. Prévost; H. Liang; J. P. Rabe; R. Schweins; J. Gummel, Langmuir 2010,
26, in
press (doi: 10.1021/la903087p).
- A. M. Bivigou-Koumba; E.
Görnitz; A.
Laschewsky; P. Müller-Buschbaum; C. M. Papadakis, Colloid Polym. Sci. 2010,
288, online first.
Preparation of non-spherical composite
nanoparticles
Alex M. van
Herk, Hans Heuts, Syed Imran Ali
Eindhoven University of Technology, The Netherlands
Colloidal nanocomposites are of major
interest to improve properties of polymeric films. Many projects focus
on incorporation of clay platelets in latex systems. Only few systems
actually succeed in encapsulation of clay platelets. In order to use
the full potential of incorporation of clay in latex particles the
orientation of the clay platelets in the final film should be
non-random. This means that the anisotropy of the clay platelet should
be expressed also in the shape of the latex particle. So peanut shaped
and flat latex particles are desired structures that in principle could
lead to maximum usage of the high aspect ratio of the clay platelets.
In this presentation the results of successful encapsulation of both
natural and synthetic clay is shown, leading to anomalous latex
particle shapes that can be used to make polymeric films with desired
alignment of the clay platelets.
Modification of the clay in combination with a designed emulsion
polymerization process is the key to obtaining the desired results.
Growing polymer from the surface by a RAFT approach can lead to uniform
layer thickness of the encapsulating polymer and therefore to flat
latex particles.
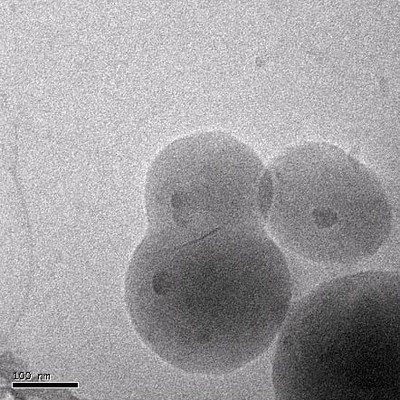 |
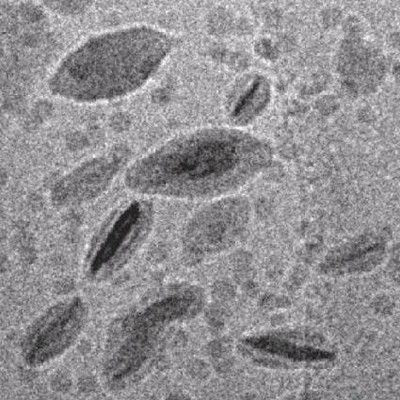 |
Dumbell
or peanut shaped latex particles obtained in a conventional starved
emulsion polymerization in the presence of modified montmorrilonite
clay platelets [1]. |
Flat
latex particles obtained in a RAFT controlled emulsion polymerization
onto synthetic Gibbsite clay platelets [3]. |
References:
- D.J. Voorn,
W. Ming, A.M. van Herk, Macromolecules 2006, 39, 4654-4656
- A.M. van Herk and A.L. German, "Microencapsulated pigments
and
fillers", contribution to the book 'Microspheres, Microcapsules
&
Liposomes', vol 1 : Preparation & Chemical Applications, Citus
Books, London , ed Prof. R Arshady (1999).
- S.I. Ali, J.P.A. Heuts, B.S. Hawkett, A.M. van Herk,
Langmuir 2009, doi:101021/1a9012697
Siden oppdatert 29.1.2010